Translate this page into:
Association of Hypoxia-Inducible Factor-1A Gene Polymorphisms and Infertility: A Case-Control Study from Northwest India
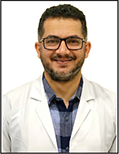
*Corresponding author: IMS Sandhu, Department of Genetics, Sri Guru Ram Das Institute of Medical Sciences and Research, SGRDUHS, Amritsar, India imssandhu@gmail.com
-
Received: ,
Accepted: ,
How to cite this article: Kaur S, Sandhu IMS, Thaman RG, Sood R, Sambyal V. Association of Hypoxia-Inducible Factor-1A Gene Polymorphisms and Infertility: A Case-Control Study from Northwest India. Fertil Sci Res. 2025;12:6. doi: 10.25259/FSR_40_2024
Abstract
Objectives
Infertility is a worldwide health problem, with one in six couples suffering from it and posing a major economic burden on the global healthcare industry. Oxidative stress is a condition depicted as an increased rate of cellular damage persuaded by oxygen-derived oxidants, mainly known as reactive oxygen species. It plays an important role in the pathophysiology of male and female infertility. Reactive oxygen species instigate a significant role in the hypoxia pathway by activating hypoxia-inducible factor-1a. In the present study, the three polymorphisms of HIF1A c.1744C>T, c.1762G>A, and c.83C>A were evaluated to find their interrelation with infertility in patients from northwest India.
Material and Methods
A total of 400 participants, including 200 infertile individuals (100 couples) as cases and 200 healthy fertile controls, were enrolled for this study. The polymerase chain reaction-restriction fragment length polymorphism method was used to assess the genotypes.
Results
For the c.1744C>T polymorphism, the frequency of CC, CT, and TT genotypes among cases and controls was found to be slightly different, but all the cases and controls had the wild-type CC genotype of c.83C>A and the GG genotype of the c.1762G>A polymorphism of HIF1A.
Conclusion
The three HIF1A polymorphisms (c.1744C>T, c.1762G>A, c.83C>A) showed no association with infertility risk in northwest Indian patients.
Keywords
Female infertility
Genetic association studies
Hypoxia inducible factor 1A subunit
Male infertility
Oxidative stress
Single nucleotide polymorphisms
INTRODUCTION
Infertility is elucidated as a disease of the reproductive system with an inability to achieve a clinical pregnancy after 1 year of regular unprotected intercourse. Infertility can also be defined as a condition in which the person’s capability to reproduce is impaired either as an individual or with his/her partner.[1] Though the accurate global prevalence rate of infertility is not known, 8%–12% of couples are estimated to be affected by infertility.[2] In the global trend, infertility affects around 186 million people, and out of this, primary infertility makes the major portion.[3] In the Indian population, the prevalence of primary infertility ranges from 3.9% to 16.8%.[4] The main causes of female infertility include endometriosis, premature ovarian insufficiency, uterine fibroids, and polycystic ovary syndrome (PCOS), while testicular deficiency is the major cause of male infertility. The factors that could influence infertility in both sexes include systemic diseases, hypogonadism, hyperprolactinemia, and various infections.[5]
Oxidative stress (OS) is an abnormal oxidation status in cells, and it has been associated with many chronic diseases, such as neurological diseases, PCOS, diabetes, cancer, and cardiovascular diseases.[6] The reactive oxygen species (ROS) produced by increased oxidative stress have been one of the important factors in the etiopathogenesis of pregnancy and affect reproduction in females. OS has a detrimental effect on oocytes, impeding their fertilisation potential. OS also triggers numerous developmental abnormalities and is estimated to be one of the major reasons for spontaneous and recurrent miscarriage in females.[7] OS is also reported to be one of the major causes of the defective functioning of sperm, leading to male infertility.[8] Spermatozoa are more vulnerable to oxidative stress due to their constrained antioxidant capacity. As a result of consequent damage to proteins and lipids in the plasma membrane of sperm due to oxidative stress, the integrity of sperm DNA is disrupted, thereby limiting the potential of a sperm to fertilise an ova.[9] ROS generated from increased oxidative stress plays a significant role in hypoxia pathways. The cellular and systemic hypoxic adaptation in mammals is predominantly regulated by hypoxia-induced factors (HIFs).[10] Hypoxia inducible factor-1 (HIF-1) is a key regulator of several genes which encode proteins involved in important processes like cell survival and proliferation, glucose and iron metabolism, and mechanisms of angiogenesis. HIF-1 is a heterodimeric transcription factor that comprises a and β subunits. The β subunit is expressed constitutively or is always ‘ON’ despite environmental conditions. The a subunit is regulated by oxygen tension, and it controls the activity of HIF-1.[11]
Hypoxia inducible factor-1a (HIF1A) gene (OMIM 603348) is mapped to the long arm of chromosome 14 region 2 band 3 sub-band 2 (14q23.2), and it consists of 15 Exons.[11] The HIF1A gene is highly polymorphic, and there are 35 single nucleotide polymorphisms (SNPs) which have been located with this gene. Out of these 35 SNPs, only 3 are located in coding regions, one in Exon 2 and the others in Exon 12. Among these, three non-synonymous polymorphisms have been reported to be associated with variant expression of HIF1A.[12] The two SNPs located within Exon 12 (codons 582 and 588) are located within the oxygen-dependent degradation domain and have been associated with transcriptional activity. The C to T transition at nucleotide 1772 leads to an amino acid change of proline to serine at codon 582 (c.1744C>T/p.P582S/rs11549465), and the G to A nucleotide substitution at point 1790 gives rise to an alanine to threonine variation at codon 588 (c.1762G>A/p.A588T/rs11549467). Another SNP located in Exon 2 at codon 28, the c.83C>A nucleotide substitution, leads to a change from serine to tyrosine at codon 28 (c.83C>A/p.S28Y/rs779897997) and lies within the critical region of the basic-helix-loop-helix (bHLH) domain in Exon 2 of the HIF1A gene. These polymorphisms are present in the critical regulatory domains of the HIF gene and might end up in the overexpression of protein, altering the expression of downstream target genes.[13] The role of the c.1744C>T polymorphism in Exon 12 of the HIF1A gene has been observed in prostate cancer and inflammation due to a hypoxic response.[14]
There have been reported associations of these polymorphisms with different diseases like cancers, coronary artery diseases, and preeclampsia.[15] The association of three nonsynonymous polymorphisms of HIF1A (c.1744C>T, c.1762G>A, c.83C>A) with infertility has not been investigated earlier but has been investigated individually with cancer susceptibility and prognosis. However, in the Mexican population, there was no association found between c.1744C>T polymorphism of HIF1A and the development of preeclampsia.[16]
There are no reported studies on the role of HIF1A polymorphisms and infertility. In the present case-control study, we estimated the association of c.1744C>T, c.1762G>A, and c.83C>A polymorphisms of HIF1A with infertility risk.
MATERIAL AND METHODS
The present case-control study was undertaken after the due approval by the Institutional Ethics Committee (Ref no. Patho 927/2018, dated 03/11/2018). The sampling of infertile patients was done from the different hospitals and infertility clinics in northwest India. A total of 200 infertile individuals (100 couples) as cases and 200 (97 males and 103 females) unrelated, age- and gender-matched, fertile control individuals were included in the study. The inclusion criteria for the cases and controls were clinically confirmed infertile patients with no offspring and individuals having children conceived by natural methods, respectively. Cases with severe autoimmune disorders such as rheumatoid arthritis, systemic lupus erythematosus, etc., were excluded from the study. For controls, the exclusion criterion was individuals conceived with the help of any assisted reproductive technologies, such as intrauterine insemination or in-vitro fertilisation.
After explaining and obtaining written informed consent of the participants, a venous blood sample of 2 ml was obtained and transferred to ethylenediaminetetraacetic acid (EDTA) vials to avoid coagulation. The samples were transported to a laboratory in an icebox and kept at -20°C until the DNA had been extracted. The standard phenol-chloroform method[17] was used to extract genomic DNA from blood samples. Published primer sequences[18] were used to screen c.83C>A, c.1744C>T, and c.1762G>A polymorphisms of HIF1A by polymerase chain reaction-restriction fragment length polymorphism (PCR-RFLP) method. To monitor the contamination, a negative control without a DNA template was incorporated in every reaction. Genotyping was performed with blinded case/control status to ensure quality control. The analysis of polymorphisms was done using PCR-RFLP as per the protocol by Sharma et al.[15] [Tables 1 and 2].
Gene (chromosomal location) | Polymorphism (RefSNP) | Location | Primers | Reference |
HIF1A (14q23.2) | p.S28Y (rs779897997) c.83C>A |
Exon 2 |
Forward-5’GGATAAGTTCTGAACGTCGA-3’ Reverse-5’-ATCCAGAAGTTTCCTCACAC-3’ |
Apaydin et al. [18] |
p.P582S (rs11549465) c.1744C>T |
Exon 12 |
Forward-5’AAGGTGTGGCCATTGTAAAAACTC-3’ Reverse-5’-GCACTAGTAGTTTCTTTATGTATG-3’ |
||
p.A588T (rs11549467) c.1762G>A |
Forward-5’-AAGGTGTGGCCATTGTAAAAACTC-3’ Reverse-5’-GCACTAGTAGTTTCTTTATGTATG-3’ |
Gene | Polymorphism (RefSNP) | PCR product size (bp) | Restriction enzyme | Incubation temperature | Allele | Expected fragment size (bp) |
HIF1A | p.S28Y (rs779897997) c.83C>A |
187 | BglII | 37°C | C A |
44, 143, 187 |
p.P582S (rs11549465) c.1744C>T |
346 | HphI | 37°C | C T |
228, 118, 346 | |
p.A588T (rs11549467) c.1762G>A |
346 | AciI | 37°C | G A |
201, 145, 346 |
SNP: Single nucleotide polymorphism; PCR: Polymerase chain reaction; bp: Base pairs.
Statistical Analysis
A statistical analysis was carried out to evaluate the association between screened polymorphisms and infertility. A two-tailed Student’s t-test was used to evaluate dissimilarities between continuous variables. The chi-square (χ2) test was used to test Hardy-Weinberg Equilibrium (HWE) by comparing the observed to expected genotype frequencies in infertile patients and controls. A significant difference in allele and genotype frequencies between the infertile patients and healthy controls was also demonstrated by using the chi-square (χ2) test. The odds ratios (OR) with a 95% confidence interval (CI) were estimated to calculate the relative risk of infertility for each genotype and allele. A value was considered statistically significant only if p<0.05. SPSS (Version 16, SPSS Inc., Chicago, IL) was used to calculate all the statistical values.
RESULTS
Characteristics of Study Participants
Out of a total of 400 participants, there were 100 infertile couples (100 males and 100 females), and 200 controls comprised 97 males and 103 females who were age- and gender-matched to infertile participants. The mean age of infertile participants was 31.61 ± 4.56 years, whereas the mean age of controls was 31.82 ± 5.68 years. Almost 75% of cases and 62% of controls were from urban backgrounds. 38% of cases and 57% of controls were vegetarian in their food choices. 33% of the cases and 17% of controls were taking alcohol regularly. While 3.5% of cases were smokers, in controls, only 0.5% were smokers. 81% of patients were diagnosed with primary infertility, and 19% of patients were diagnosed with secondary infertility (nulliparous) [Table 3]. Conditions such as PCOS, ovarian failure, secondary amenorrhoea, hyperprolactinemia, teratozoospermia, oligozoospermia, etc., are clinically listed as some of the causes of infertility for female & male cases. Detailed demographic data is published and available.[19]
Characteristics | Cases n(%) | Controls n(%) | p-value |
Age (years) | |||
Mean ± SD | 31.61 ± 4.56 | 31.82 ± 5.68 | - |
Habitat | |||
Urban | 149 (74.5) | 124 (62.0) | 0.0075# |
Rural | 51 (25.5) | 76 (38.0) | |
Diet | |||
Vegetarian | 76 (38.0) | 113 (56.5) | |
Nonvegetarian | 124 (62.0) | 87 (43.5) | 0.0002# |
Alcohol drinking | |||
Drinkers | 66 (33.0) | 33 (16.5) | 0.0002# |
Nondrinkers | 134 (67.0) | 167 (83.5) | |
Smoking | |||
Smokers | 7 (3.5) | 1 (0.5) | NC |
Nonsmokers | 193 (96.5) | 199 (99.5) | |
Body mass index (BMI)@ kg/m2 | |||
Combined BMI (Mean ± SD) | 26.04 ± 4.70 | 24.82 ± 2.94 | 0.001** |
Type of infertility | |||
Primary | 162 (81.0) | - | |
Secondary | 38 (19.0) | - | - |
#p-values calculated using χ2 test;
**p-values calculated using t-test; n: Number of participants; NC: Not calculated; %: Percentage frequency; SD: Standard deviation.
Association Between HIF1A Polymorphisms and Infertility
The allelic and genotypic frequencies of HIF1A polymorphisms c.1762G>A, c.83C>A, and c.1744C>T in the cases and controls are shown in Table 4. All the cases and controls had only the wild type (CC) genotype for c.83C>A polymorphism and wild type (GG) genotype for c.1762G>A polymorphism. For c.1744C>T polymorphism, the frequency of CC, CT, and TT genotypes among the cases and controls was 75.0 vs. 69.0%, 20.5 vs. 28.0%, and 4.5 vs. 3.0%, respectively [Table 4]. A slightly increased frequency of CT genotype was observed in controls (28%) as compared to infertile patients (20.5%). No statistically significant difference was observed for the C and T allele frequencies between the cases and the controls (p > 0.05). When the polymorphism data for c.1744C>T was separately analyzed for males and females in cases and controls, it showed no statistically significant difference [Table 4].
Variant | Genotype | Genotype/Allele | Cases n(%) | Controls n(%) | SAS reference population allele frequencies (%) (dbSNP database) | OR (95% CI) | p-value |
c.83C>A (rs779897997) |
Genotype | CC | 200(100) | 200(100) | |||
CA | - | - | |||||
AA | - | - | NC | ||||
Allele | NC | ||||||
C | 400 (100) | 400 (100) | 100 | ||||
A | - | - | 0.00 | ||||
c.1744C>T (rs11549465) |
Genotype (Combined) | CC | 150 (75.0) | 138 (69.0) | 1 (reference) | ||
CT | 41 (20.5) | 56 (28.0) | 0.67(0.42–1.07) | ||||
TT | 09 (4.5) | 06 (3.0) | 1.38(0.48–3.98) | 0.095 | |||
Allele | |||||||
C | 341 (85.25) | 332 (83) | 89.21 | 1 (reference) | 0.551 | ||
T | 59 (14.75) | 68 (17) | 10.79 | 0.89 (0.61–1.31) | 0.57 | ||
Genotype (Males) |
CC | 70 (70) | 64 (65.9) | 1(reference) | |||
CT | 26 (26) | 29 (29.9) | 0.81(0.43-1.53) | 0.53 | |||
TT | 04 (04.0) | 04 (4.2) | 0.91 (0.21–3.80) | 0.90 | |||
Allele | |||||||
C | 166 (83.0) | 157 (80.9) | 1 (reference) | ||||
T | 34 (17.0) | 37 (19.1) | 0.87 (0.52-1.45) | 0.59 | |||
Genotype (females) |
CC | 80 (80.0) | 74 (71.8) | 1 (reference) | |||
CT | 15 (15.0) | 27 (26.2) | 0.51 (0.25–1.04) | 0.06 | |||
TT | 5 (5.0) | 02 (2.0) | 2.31 (0.43–12.28) | 0.32 | |||
Allele | |||||||
C | 175 (87.5) | 175 (84.9) | 1 (reference) | ||||
T | 25 (12.5) | 31 (15.0) | 0.81 (0.46–1.42) | 0.45 | |||
c.1762G>A (rs11549467) |
Genotype | GG | 200 (100) | 200 (100) | |||
GA | - | - | |||||
AA | - | - | NC | NC | |||
Allele | |||||||
G | 400 (100) | 400 (100) | 99.7 | ||||
A | - | - | 0.3 |
NC: Not calculated; OR: Odds ratio; CI: Confidence intervals; SNP: Single nucleotide polymorphism; CI: Confidence interval; A: Adenine; T: Thymine; G: Guanine; C: Cytosine; NC: Not calculated; dbSNP: Single nucleotide polymorphism database.
The comparison of allele frequencies between the studied population and the SAS (South Asian) reference population data revealed interesting insights. The study’s allele frequencies for specific variants were evaluated against the data retrieved from the dbSNP database. Interestingly, all the variants showed complete concordance with the SAS reference population allele frequencies, suggesting that there was no population bias in the present study. The inclusion of SAS reference data provides a robust baseline for evaluating the distinct genetic profiles of the studied population [Table 4].
DISCUSSION
Infertility is increasing and has turned out to be a global problem affecting human development. It is, therefore, imperative to further study the molecular mechanisms of infertility and search for molecular targets to reduce the burden of disease.[20] The corroboration of the causal association between hypoxia and infertility (male/female) may help provide more options for the treatment of infertility. Exposure of male rats to chronic hypoxia and intermittent hypoxia has been reported to result in a change in testicular morphology and loss of spermatogenic cells in all stages of the spermatogenic cycle.[21] The production of testosterone in males is also oxygen tension controlled.[22] Testosterone is a potent paracrine factor for spermatogenesis, and prolonged exposure to hypoxia results in low sperm count and infertility.[22] In females, during the development or progression of endometriosis, hypoxia causes epigenome reprogramming. It happens by stimulating the degradation of DNA methyltransferase 1 mRNA, which alters the expression profile of some crucial genes responsible for endometriosis. This also indicates that hypoxia may contribute to endometriosis using gene regulation.[23]
Various complex diseases could be a result of individual DNA sequence variations, which are known as genetic polymorphisms. Among these variations, SNPs are the most commonly occurring genetic alterations. Gene expression or protein function can be affected by functional polymorphisms, which are present in gene regulatory or coding sequences.[24] HIF1A gene SNPs are more frequent in several cancers than in healthy groups.[25]
The cellular O2 concentrations control the expression of HIF1A. Any changes in HIF1A expression can lead to spontaneous abortion, recurrent pregnancy loss, and unexplained infertility in females. ROS production due to oxidative stress and excessive ROS formation generates an environment unsuitable for normal female physiological reactions that can lead to reproductive disease and unexplained infertility.[26] In the human placenta, HIF1A is the major transducer of hypoxia signaling.[16] An aberrant expression of HIF1A with an increased number and volume of micro blood vessels in the peri-implantation endometrium of women with recurrent miscarriages has been reported.[27] Hypoxia directly damages the testicular seminiferous tubule, causing seminiferous epithelium dysfunction and spermatogenic cell loss in males. Hypoxia can also cause spermatogenic cells to lose their equilibrium between oxidative phosphorylation and glycolysis, resulting in spermatogonia self-renewal and differentiation, as well as meiosis failure. Hypoxia also causes spermatogenic arrest and erectile dysfunction by disrupting the release of reproductive hormones.[20]
A change in the base of C to T at 1772 in Exon 12 of the HIF1A gene results in an elevation of its transcriptional activity as in comparison to the wild-type isoform and have the potential as a genetic marker for tumour prognosis.[28] The frequency of the c.1744C>T polymorphism of the HIF1A gene was reported to be very low and was not associated with the development of preeclampsia in the Mexican population.[16] In the present study, for HIF1A c.1744C>T, the frequency of CC, CT, and TT genotypes in infertile patients was 75%, 20.5%, and 4.5%, respectively. The frequency of CC, CT, and TT genotypes in fertile controls was 69%, 28%, and 3%, respectively. There was no significant difference in the heterozygous variant CT allele frequency and the homozygous variant TT allele frequency of patients and controls. These results are similar to a previous report from the northwest Indian population in breast cancer c.1744C>T, which has shown no significant difference (p > 0.05) in genotype and allele frequencies of the HIF-1a c.1744C>T polymorphism between cases and control individuals.[15] Activation of HIF1A as a gain of function mechanism, which is driven by stabilisation of HIF1A mRNA, has been caused by the c.1744C>T (p.P582S) polymorphism and has been linked as a risk factor for cancer in Asian origin females[29] and to increased risk of cancer metastasis.[30]
For c.1762G>A (p.A588T) polymorphisms, only homozygous wild-type GG genotype was observed in all individuals in the present study. Similar findings were also reported in previous reports for breast cancer.[18,28,31] A significant association was demonstrated between the ‘A’ allele of the c.1762G>A polymorphism and the probability of cancer risk in various cancers like pancreatic, lung, renal, head, and neck but not in breast and prostate cancer.[32]
As for the c.83C>A polymorphism of the HIF1A gene, which leads to the substitution of the amino acid serine by tyrosine at codon 28 of Exon 2 and lies in the basic helix-loop-helix (bHLH) domain of the HIF1A protein, all the infertile cases and fertile controls were found to be homozygous wild-type CC genotype in the present study. There have been no previous reports of the c.83C>A polymorphism in infertility. However, the distribution of the c.83C>A polymorphism has been consistent with previous reports in breast cancer patients in northwest India.[15]
Similar to the results of the present study, the variant ‘A’ allele of c.83C>A and ‘A’ allele of c.1762G>A polymorphisms were not observed in Turkish breast cancer patients.[18] In breast cancer patients from the Turkish population and in Caucasian ovarian, cervical, and endometrial cancer patients, c.1762G>A and c.83C>A polymorphism variants have not been observed.[18,33]
A study on the Malaysian population observed a statistically non-significant association of the allele ‘A’ of the c.83C>A and c.1762G>A polymorphisms in breast cancer patients and controls, respectively.[28]A non-statistically significant association was observed in Korean breast cancer patients for c.1762G>A polymorphism variants.[31] Some studies on Indian populations on other gene polymorphisms, such as FSHR and AMH, have shown a significant association between these polymorphisms and chances of pregnancy and its outcomes.[34–36] The results from the present study are quite similar to the previously reported results from Caucasian populations and are in direct contrast to the results from the population from East Asia. The reason behind this may be that our study population is ethnically a mixture of Indo-Scythian and Caucasian populations,[37] and this is a unique feature of the population from northwest India, especially the Punjab region.[38]
A major limitation of the study is that male and female causes of infertility have not been separately analysed for their association with HIF1A. Future work must analyse well-defined cases of male and female infertility individually for their association with genetic variants of HIF1A before concluding the involvement of this gene in infertility.
CONCLUSION
The present study has not found any association between the polymorphic variants of HIF1A and infertility in the study sample from northwest India. The present study findings need to be validated using a larger sample size from various ethnic groups living in different regions of India. In future, the other polymorphisms of HIF1A, HIF-1β, HIF-2a, and HIF-3a can be explored for their association with infertility.
Author contribution
SK, RGT, RS and VS: Conception and design of study; SK: Literature search and compilation; SK and IMSS: Carried out population screening, and sample collection, DNA isolation, and genotyping of the samples; SK: Statistical analyses and interpretation of data; RS: Was the clinical collaborators who evaluated the cases and samples. All authors contributed in drafting, revising and critically reviewing the article, and have given final approval of the version to be published.
Ethical approval
The research/study was approved by the Institutional Review Board at Sri Guru Ram Das Institute of Medical Sciences & Research, number Patho927/2018, dated 03/11/2018.
Declaration of patient consent
The authors certify that they have obtained all appropriate patient consent.
Financial support and sponsorship
Nil.
Conflicts of interest
There are no conflicts of interest.
Use of artificial intelligence (AI)-assisted technology for manuscript preparation
The authors confirm that there was no use of artificial intelligence (AI)-assisted technology for assisting in the writing or editing of the manuscript and no images were manipulated using AI.
REFERENCES
- Fertility and infertility: Definition and Epidemiology. Clin Biochem. 2018;62:2-10.
- [CrossRef] [PubMed] [Google Scholar]
- Infertility: An International Health Problem. Int J Gynaecol Obstet. 1994;46:155-63.
- [CrossRef] [PubMed] [Google Scholar]
- Infertility Around the Globe: New Thinking on Gender, Reproductive Technologies and Global Movements in the 21st Century. Hum Reprod Update. 2015;21:411-26.
- [CrossRef] [PubMed] [Google Scholar]
- Role of Genetics, Environmental, and Lifestyle Factors Responsible for Infertility—A Review. Fert Sci Res. 2023;10:131-8.
- [CrossRef] [Google Scholar]
- Oxidative Stress: Major Executioner in Disease Pathology, Role in Sperm DNA Damage and Preventive Strategies. Front Biosci (Schol Ed). 2017;9:420-47.
- [CrossRef] [Google Scholar]
- Expression of Hypoxia-Inducible Factor 1: Mechanisms and Consequences. Biochem Pharmacol. 2000;59:47-53.
- [CrossRef] [PubMed] [Google Scholar]
- The Role of Oxidative Stress in Female Infertility and in Vitro Fertilization. Postepy Hig Med Dosw (Online). 2017;71:359-66.
- [CrossRef] [PubMed] [Google Scholar]
- Rate of de novo Mutations and the Importance of Father’s Age to Disease Risk. Nature. 2012;488:471-5.
- [CrossRef] [PubMed] [PubMed Central] [Google Scholar]
- Oxidative Stress and Male Reproductive Health. Asian J Androl. 2014;16:31-8.
- [CrossRef] [PubMed] [PubMed Central] [Google Scholar]
- Hypoxia-Inducible Factors: Coupling Glucose Metabolism and Redox Regulation With Induction of the Breast Cancer Stem Cell Phenotype. EMBO J. 2017;36:252-9.
- [CrossRef] [PubMed] [PubMed Central] [Google Scholar]
- Targeting HIF-1 for Cancer Therapy. Nat Rev Cancer. 2003;3:721-32.
- [CrossRef] [PubMed] [Google Scholar]
- Genetic Variation in the Hypoxia-Inducible Factor-1alpha Gene is Associated With Type 2 Diabetes in Japanese. J Clin Endocrinol Metab. 2005;90:5841-7.
- [CrossRef] [PubMed] [Google Scholar]
- Polymorphism in the Hypoxia-Inducible Factor 1alpha Gene May Confer Susceptibility to Androgen-Independent Prostate Cancer. Cancer Biol Ther. 2005;4:1222-5.
- [CrossRef] [PubMed] [Google Scholar]
- The HIF-1alpha C1772T Polymorphism May be Associated with Susceptibility to Clinically Localised Prostate Cancer But Not With Elevated Expression of Hypoxic Biomarkers. Cancer Biol Ther. 2009;8:118-24.
- [CrossRef] [PubMed] [Google Scholar]
- No Association of Hypoxia Inducible Factor-1alpha Gene Polymorphisms With Breast Cancer in North-West Indians. Asian Pac J Cancer Prev. 2014;15:9973-8.
- [CrossRef] [PubMed] [Google Scholar]
- Polymorphisms in the Hypoxia-Inducible Factor 1 Alpha Gene In Mexican Patients With Preeclampsia: A Case-Control Study. BMC Res Notes. 2011;4:68.
- [CrossRef] [PubMed] [PubMed Central] [Google Scholar]
- Rapid Purification of Human DNA From Whole Blood for Potential Application in Clinical Chemistry Laboratories. Clin Chem. 1990;36:261-4.
- [PubMed] [Google Scholar]
- Single Nucleotide Polymorphisms in the Hypoxia-Inducible Factor-1alpha (Hif-1alpha) Gene in Human Sporadic Breast Cancer. Arch Med Res. 2008;39:338-45.
- [CrossRef] [PubMed] [Google Scholar]
- Comparison of Demographic, Socioeconomic, and Lifestyle Determinants in Infertile and Fertile Males and Females From Two Big Cities of Punjab. BLDE Univ J Health Sci. 2022;7:294-301.
- [Google Scholar]
- Effects of Environmental and Pathological Hypoxia on Male Fertility. Front Cell Dev Biol. 2021;9:725933.
- [CrossRef] [PubMed] [PubMed Central] [Google Scholar]
- Effects of Chronic Hypobaric Hypoxia on Testis Histology and Round Spermatid Oxidative Metabolism. Andrologia. 2005;37:47-52.
- [CrossRef] [PubMed] [Google Scholar]
- Effect of Normobaric Hypoxia on the Testis in a Murine Model. Andrologia. 2013;45:332-8.
- [CrossRef] [PubMed] [Google Scholar]
- Coordination of AUF1 and miR-148a Destabilizes DNA Methyltransferase 1 mRNA Under Hypoxia in Endometriosis. Mol Hum Reprod. 2015;21:894-904.
- [CrossRef] [PubMed] [Google Scholar]
- Finding Genes Influencing Susceptibility to Complex Diseases in the Post-Genome Era. Am J Pharmacogenomics. 2001;1:203-21.
- [CrossRef] [PubMed] [Google Scholar]
- Association of the Hypoxia-Inducible Factor-1alpha (Hif-1alpha) Gene Polymorphisms With Prognosis in Ovarian Clear Cell Carcinoma. J Ovarian Res. 2019;12:7.
- [CrossRef] [PubMed] [PubMed Central] [Google Scholar]
- The Effects of Oxidative Stress on Female Reproduction: A Review. Reprod Biol Endocrinol. 2012;10:49.
- [Google Scholar]
- Hypoxia Inducible Factor and Microvessels in Peri-Implantation Endometrium of Women With Recurrent Miscarriage. Fertil Steril. 2016;105:1496-502e4.
- [CrossRef] [PubMed] [Google Scholar]
- Associations Between Hypoxia-Inducible Factor-1alpha (HIF-1alpha) Gene Polymorphisms and Risk of Developing Breast Cancer. Neoplasma. 2009;56:441-7.
- [CrossRef] [PubMed] [Google Scholar]
- The Association Between Hypoxia-Inducible Factor-1 Alpha Gene C1772T Polymorphism and Cancer Risk: A Meta-Analysis of 37 Case-Control Studies. PLoS One. 2013;8:e83441.
- [CrossRef] [PubMed] [PubMed Central] [Google Scholar]
- Hypoxia-Inducible Factor-1alpha Polymorphisms and Risk of Cancer Metastasis: A Meta-Analysis. PLoS One. 2013;8:e70961.
- [CrossRef] [PubMed] [PubMed Central] [Google Scholar]
- The C1772T Genetic Polymorphism in Human HIF-1alpha Gene Associates With Expression of HIF-1alpha Protein in Breast Cancer. Oncol Rep. 2008;205:1181-7.
- [PubMed] [Google Scholar]
- The Association Between Hypoxia-Inducible Factor-1 Alpha Gene G1790A Polymorphism and Cancer Risk: A Meta-Analysis of 28 Case-Control Studies. Cancer Cell Int. 2014;14:37.
- [CrossRef] [PubMed] [PubMed Central] [Google Scholar]
- An Investigation of Relationships Between Hypoxia-Inducible Factor-1 Alpha Gene Polymorphisms and Ovarian, Cervical and Endometrial Cancers. Cancer Detect Prev. 2007;31:102-9.
- [CrossRef] [PubMed] [Google Scholar]
- Follicle Stimulating Hormone Receptor Gene Variants in Women With Primary and Secondary Amenorrhea. J Assist Reprod Genet. 2010;27:317-26.
- [CrossRef] [PubMed] [PubMed Central] [Google Scholar]
- Association of AMH and AMHR2 Gene Polymorphisms With Ovarian Response and Pregnancy Outcomes in Indian Women. J Assist Reprod Genet. 2022;39:1633-42.
- [CrossRef] [PubMed] [PubMed Central] [Google Scholar]
- Poor Ovarian Response to Gonadotrophin Stimulation is Associated with FSH receptor Polymorphism. Reprod Biomed Online. 2009;18:509-15.
- [CrossRef] [PubMed] [Google Scholar]
- The Distribution of Genetical, Morphological, and Behavioural Traits Among the Peoples of Indian Region: Bangladesh, Bhutan, India, Maldives, Nepal, Pakistan, Sri Lanka. 1992
- [Google Scholar]
- Ethnic India: A Genomic View, With Special Reference to Peopling and Structure. Genome Res. 2003;13:2277-90.
- [CrossRef] [PubMed] [PubMed Central] [Google Scholar]